Published in Nature Communications, New Study Examines the Effect of Defects on the Intrinsic Strength and Stiffness of the Nanomaterial Graphene
January 23, 2014
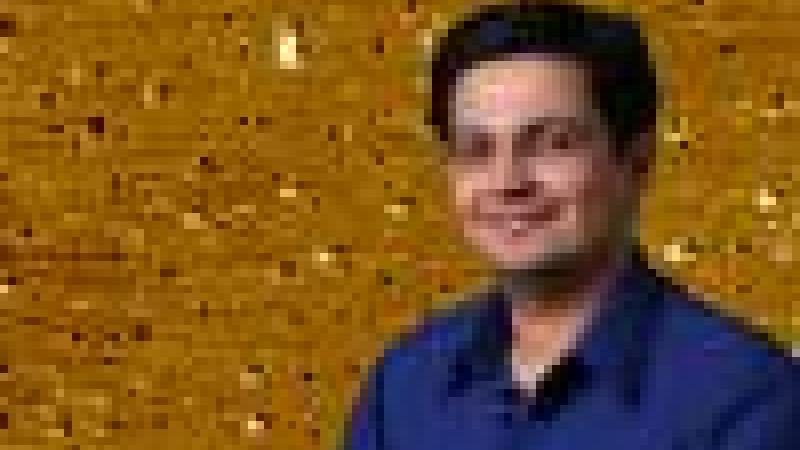
A new study led by engineers at Rensselaer Polytechnic Institute demonstrates, for the first time, a simple method for determining the strength and stiffness of practical samples of the nanomaterial graphene.
The study, published today in the journal Nature Communications, includes data that enables researchers to correlate the precise strength and stiffness of non-pristine graphene samples to their Raman signatures. Raman spectroscopy is a simple, non-contact, and non-destructive method widely used by scientists and engineers to characterize the state of defectiveness of graphene.
This fundamental research finding holds the potential to help inform the development and creation of new composite materials, nanoelectronics, and devices that take advantage of graphene’s unique mechanical properties.
“Our results provide researchers a facile, rapid, and non-destructive method to determine the strength and stiffness of graphene samples, with a high degree of accuracy” said Nikhil Koratkar, the John A. Clark and Edward T. Crossan Professor of Engineering at Rensselaer, who led the study. “This data will be immediately useful to everyone in academia, government, and industry who uses graphene in their research and product development.”
See the study, titled “Effect of defects on the Intrinsic Strength and Stiffness of Graphene,” at: http://go.nature.com/Gpy9fT
The thinnest material known to science, graphene is a single layer of carbon atoms arranged like a nanoscale chicken-wire fence. Graphene is physically strong and chemically inert, and researchers have been investigating how to leverage its unique properties to develop a range of applications including batteries, gas sensors, and computer chips.
A landmark study published in 2008 characterized the strength of a pristine layer of graphene that was isolated by using common adhesive tape to exfoliate a layer of graphene from bulk graphite (like the graphite commonly found in pencils). This method of creating graphene, however, is prohibitively time consuming and not suitable for producing large quantities.
Other processes used to create large quantities of graphene, including chemical vapor deposition and oxidizing graphite, result in graphene layers with holes, voids, leftover oxygen atoms, and other defects. In this new study, Koratkar and the research team investigated how the density and type of these defects affect the mechanical properties of such practical graphene samples that are most commonly used in academic, industrial, and government laboratories.
The research team created samples of graphene with a range of densities and types of defects, and then characterized the samples using Raman spectroscopy. The team then used a nanoindentation technique to press sharp diamond tips into the graphene samples, and recorded how the samples stretched and, in some cases, tore.
Koratkar said he was surprised by the finding that graphene is able to retain its outstanding mechanical properties even after its surface is littered with oxygen atoms, which disrupt the graphene’s perfect sp2 carbon bonding network. This is interesting, he said, since the electrical and thermal properties of graphene are known to degrade significantly due to such defects, but this study indicates that graphene sheets are far more rugged and mechanically robust than what was previously thought. The research team used molecular dynamics simulations to help understand and interpret these observations.
The study also indicated that when holes or vacancy defects are created in the graphene structure, its strength and stiffness degrades significantly.
An important practical implication of this work is that it provides a direct relationship between the intrinsic strength and stiffness of defective graphene samples and their Raman signature.
“By establishing a relationship between the Raman-spectra of defective graphene and its mechanical properties, we have provided a simple and non-destructive methodology to identify some important mechanical properties of graphene,” Koratkar said.
Koratkar is a faculty member in the Department of Mechanical, Aerospace, and Nuclear Engineering (MANE) at Rensselaer, with a joint appointment in the Department of Materials Science and Engineering. He is affiliated with the university’s Center for Future Energy Systems and Rensselaer Nanotechnology Center.
Along with Koratkar, authors of the study are: Catalin Picu, professor in MANE at Rensselaer;
Rensselaer graduate students Ardavan Zandiatashbar and Nithin Mathew; James Hone, professor at Columbia University; Gwan-Hyoung Lee, postdoctoral researcher at Columbia; Columbia graduate students Sung Joo An and Sunwoo Lee; Mauricio Terrones, professor at Pennsylvania State University; and Takuya Hayashi, professor at Shinshu University in Japan.
This study was supported with funding from the U.S. Office of Naval Research, the National Science Foundation, the Air Force Office of Scientific Research Multidisciplinary Research Program of the University Research Initiative, and the Samsung-SKKU Graphene Center.